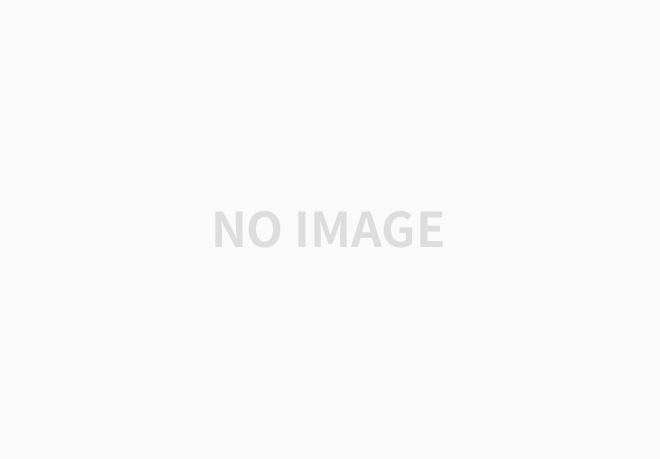
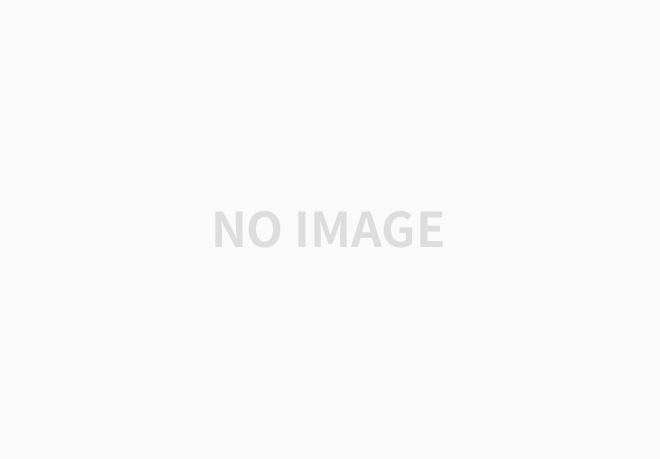
The riverbed of the Jhelum river at the powerhouse location was rised is that the width of the river became narrow due to the construction of the access bridge and newly built left bank around the bridge. Moreover, it can also be guessed that the dumped materials played an important role as the river bed was being increased by deposits, durinvg the process of the river flow channel change with the temporary cofferdam at the Jhelum river for the purpose of bridge construction in 2011.
The riverbed erosion and deposition depends on the flow discharge as well as the sediment load.
The objective of the sediment transport analysis is to presuppose the riverbed-level variation of the Jhelum river.
신광균 부장, 수력부(kgshin@samaneng.com)
1. Introduction
This sediment transport analysis of the Jhelum river was carried out according to the item 1.2.2.1 and 1.2.2.2 from the OE comments (LET/PES.ST-EPCC/966).
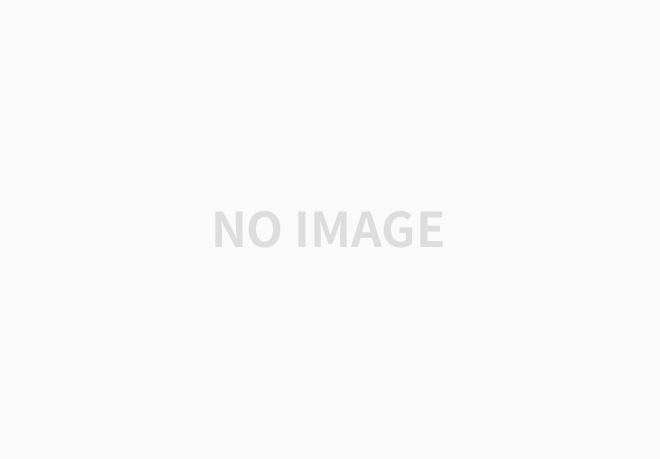
Figure 1. The Jhelum river before construction
OE was concerned about the tailwater rating curve as follows: “The latest tailwater rating of Jhelum river at powerhouse location has raised serious concerns as the bed porfile on Jhelum river in the vicinity of the powerhouse has significantly changed as compared to the bed profile surveyed at the feasibility stage.”
The reason why the riverbed of the Jhelum river at the powerhouse location was rised is that the width of the river became narrow due to the construction of the access bridge and newly built left bank around the bridge. Moreover, it can also be guessed that the dumped materials played an important role as the river bed was being increased by deposits, during the process of the river flow channel change with the temporary cofferdam at the Jhelum river for the purpose of bridge construction in 2011.
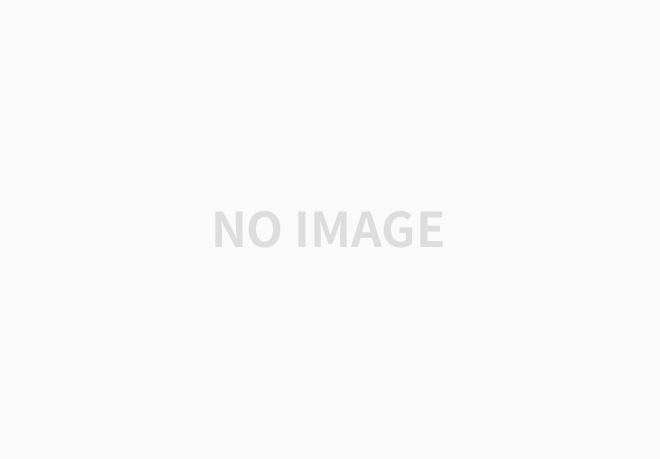
Figure 2. The view of the temporary cofferdam under construction at the end of 2011.
EPCC has developed the Dredging Plan shown in the report that was submitted in December 2014 As the power generation has been decreased due to the higher tailwater rating curve. According to the plan, riverbed will be excavated near the powerhouse up to 900m point of the downstream way. Thus, it shall lower the tailwater rating curve to the basic design level. Nonetheless, the riverbed erosion and deposition depends on the flow discharge as well as the sediment load.
The objectiove of the sediment transport analysis is to presuppose the riverbed-level variation of the Jhelum river.
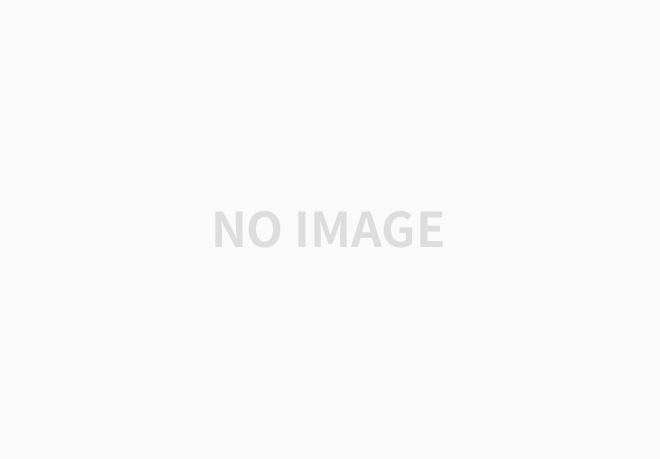
Figure 3. The latest view of the Jhelum river
2. Input Data
2.1 Flow Discharge
For the mean daily discharge of the Jhelum river, the analysis was carried out for 34 years data from 1976 to 2012. Figure 4 shows the mean daily discharge between 1976 and 2012.
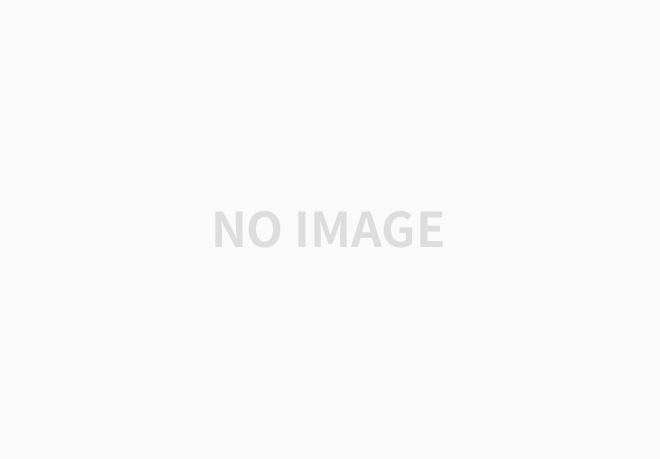
Figure 4. Mean daily discharge of Jhelum river
The HEC-RAS modelling has repeated the mean daily discharge five times in order to presuppose the riverbed variation of the Jhelum river between 2015 and 2019.
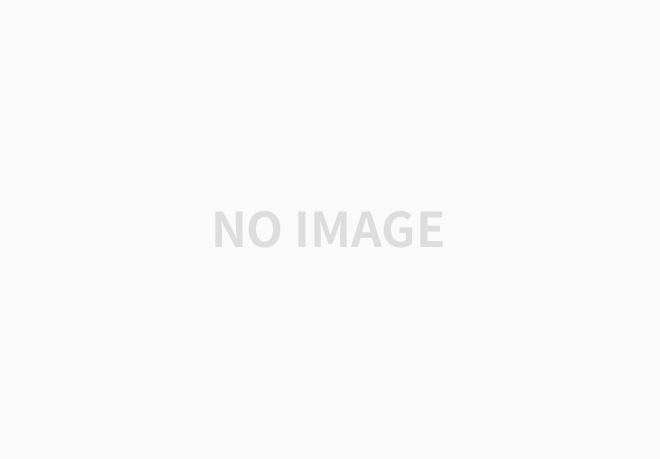
Figure 5. Flow series for Jhelum river
2.2 Sediment Load
The suspended sediment data have used the data from 1980 to 1996 at Kohala location on the Jhelum river and also the data from 1996 to 2013 at Chattar Kalas in the Jhelum river.
The equation that relates water discharges and suspended loads is as follows:
Where,
: suspended sediment load in ton per day
: water discharge in m3/sec
The equation is based on the following data:
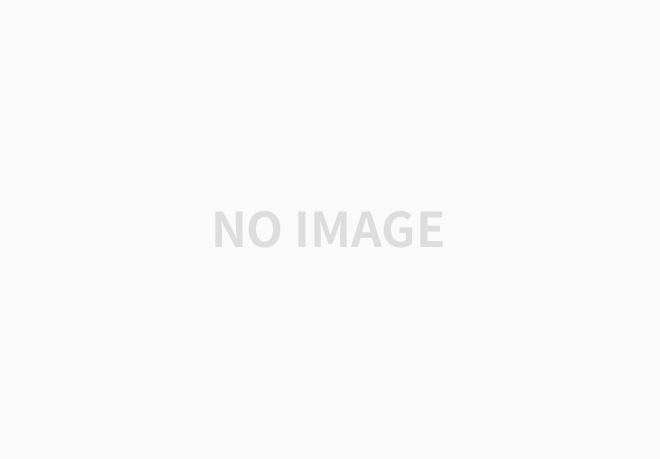
Figure 6. Suspended sediment data (Kohala 1980-199 & Chatter kalas 1996-2013)
The suspended sediment loads in the Jhelum river show relatively low tendency as compared to the suspended sediment loads in the Kunhar river.
The suspended sediment concentration in the Jhelum river was observed as a 10,900ppm on 27th July 1995 and also the average suspended sediment concentration was 369ppm. Therefore, this suspended sediment concentration was found as smaller than approximate 1,200ppm of the average suspended sediment concentration in the Kunhar river.
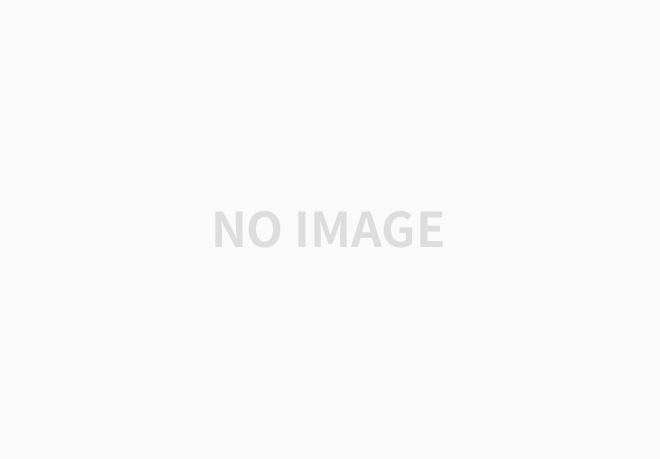
Figure 7. Sediment concentration at Jhelum river
The average composition of the suspended sediment consists of 27% of clay, 55% of silt and 17% of sand. According to this, the silt and clay ratio is high in the Jhelum river. However, depending on the discharge, sand ratio during the flood was increased up to maximum 72% on August 2010. At that time, the silt ratio was 21% and the clay was 7%. But, most of the data shows the sand ratio within 20%.
Table 1. Average measured composition of suspended sediment of the Jhelum River
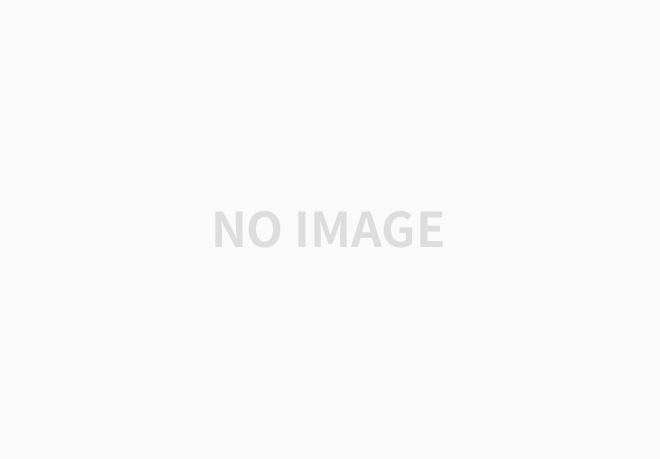
The river bed data used the sampling data of the Jhelum river from the report by Fichtner (October 2013). The mean grain size of bed material d50 is 40mm.
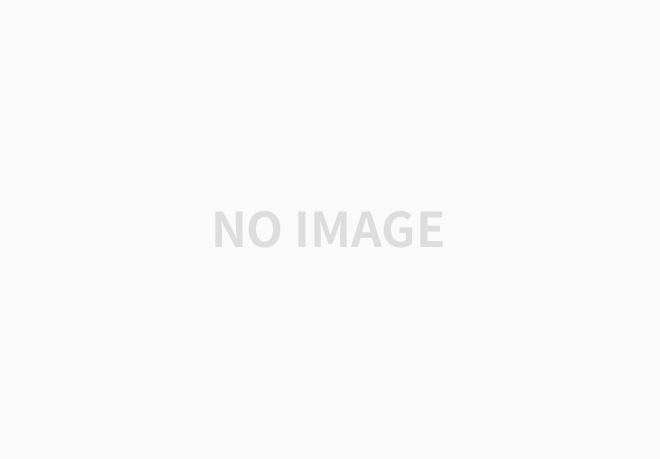
Figure 8. Gradation curve of bed material at Jhelum river
2.3 Geometry of the Jhelum River
In the Figure 9, for the pre-surveyed sections, the river survey has been carried out at total 32 points which consists of exiting 22 points as well as additional surveying points near the upstream and downstream of the powerhouse and the point about 500m toward the downstream direction.
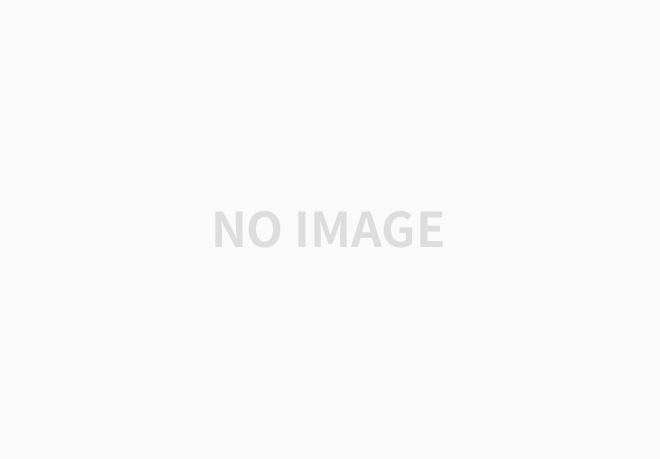
Figure 9. Location of sections
Figure 10 shows the thalweg of the surveyed sections. From No. 13 to No. 11 shows the low river bed compared to the past and this is considered that the scour was occurring at the river bed d theue to strong flow velocity to near the end of river bend. Thus, it is considered that the river section is changeable depend on the surveying time because the scour and deposition is a repeated phenomena in the river bed. After no. 10, it shows that the river bed has risen a lot compared to the past.
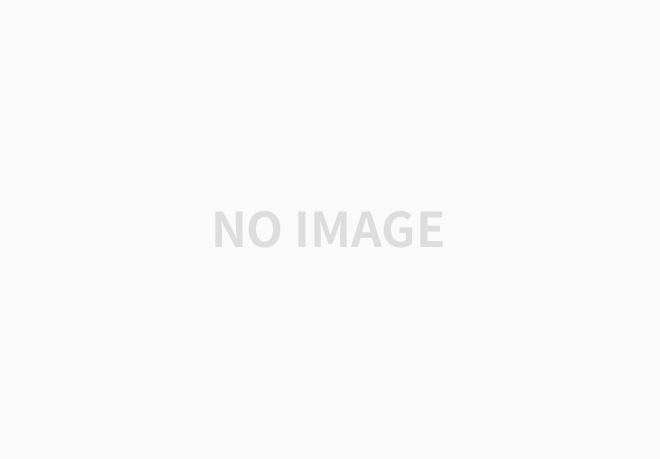
Figure 10. Bed profile of Jhelum river
Table 2 shows the comparison of the thalweg between the basic design stage and the current riverbed. According to this, the survey was carried out approximate 2km from the section No. 21 to No. -5. The bridge point is no. 4 and no.+2 is the investigated point of the tailwater level at the powerhouse.
Table 2. Longitudinal bed profile
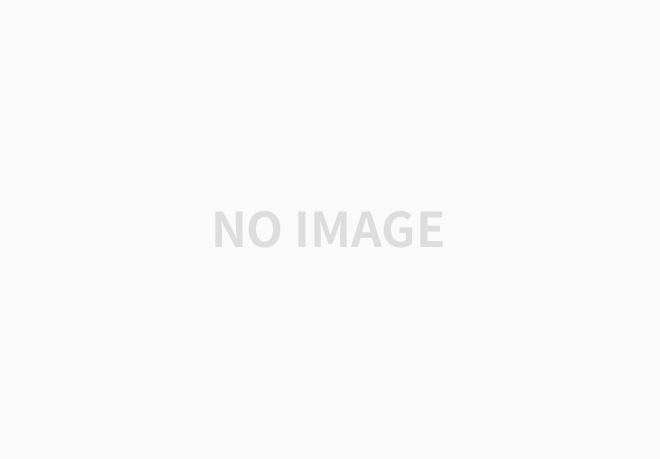
2.4 Methodology
The sediment model, HEC-RAS, requires a geometry file, a quasi-unsteady flow file, a sediment file and a sediment analysis plan file. The result presented in this report has been obtained numerical simulations with 1D model, HEC-RAS model. This model uses the current bathymetry of the Jhelum river as starting point.
There are currently seven sediment transport potential functions in HEC-RAS. The American Society of Civil Engineers (ASCE Task Committee on Relations between Morphology of Small Streams and Sediment Yield) has evaluated that the Yang equation has a relatively high accuracy. Therefore, the Yang equation (sand and gravel eqns.) has been applied to the transport function.
Yang Equation
Yang(1973, 1984) is a total load transport equation which bases transport on Stream Power, the product of velocity and shear stress. The function was developed and tested over a variety of flum and field data. The equation is composed of two separate relations for sand and gravel transport. The transition between sand-gravel is smoothed over in order to avoid large discontinuities. Yang tends to be very sensitive to stream velocity, and ot is more sensitive to fall velocity than most.
3. The sediment transport simulation at the Jhelum river by HEC-RAS
3.1 Quasi-unsteady flow
The data for the current riverbed of the Jhelum river was surveyed since end of October to the mid of November, in 2014. In the HEC-RAS modelling, it used the flow data from the mean daily discharge data of Figure 4 and created the flow data for 5 years from 1 January 2015 to 31 December 2019 (Figure 5) as an input data.
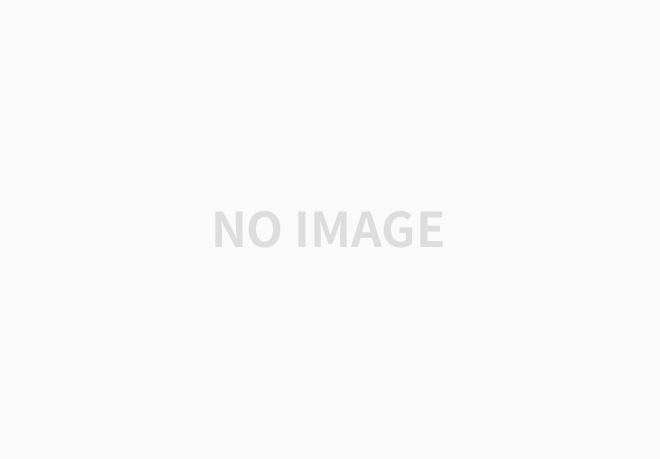
Figure 11. Flow data for HEC-RAS
As no discharge data of 2015’ of Jhelum river is available, therefore, the mean daily flow was supposed and repeated for 5 years from 2015 to 2019 (Figure 5). Even though the actual discharge may be more or less, however, the mean daily flow has been used to the modelling in order to presuppose the riverbed-level variation in the Jhelum river.
The normal depth has been applied to the initial downstream boundary condition which was 0.011608. Also, the riverbed slope value was inserted between section No. 0 and No. -5. In case of the friction slope, although it is the slope of the energy grade line, however, it has been input as the slope of the riverbed.
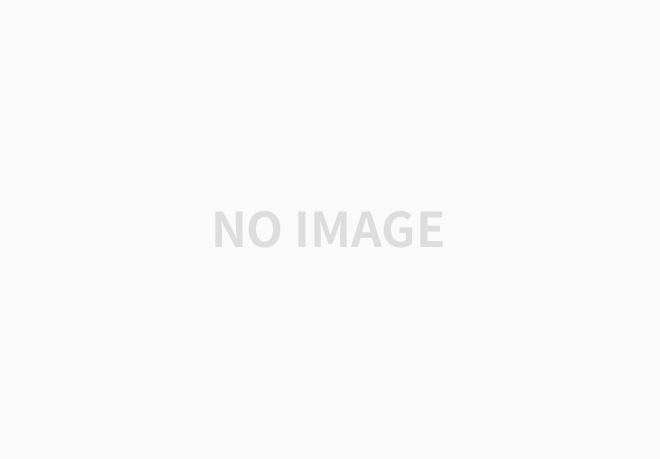
Figure 12. Downstream boundary (Friction slope)
3.2 Sediment data
Among the sediment data, the transport function used the Yang equation and also the Exner 5 and the Ruby have been applied to the sorting method and the fall velocity method, respectively.
In addition, the bed gradation has been using the data which were surveyed by Fichtner.(Figure 8)
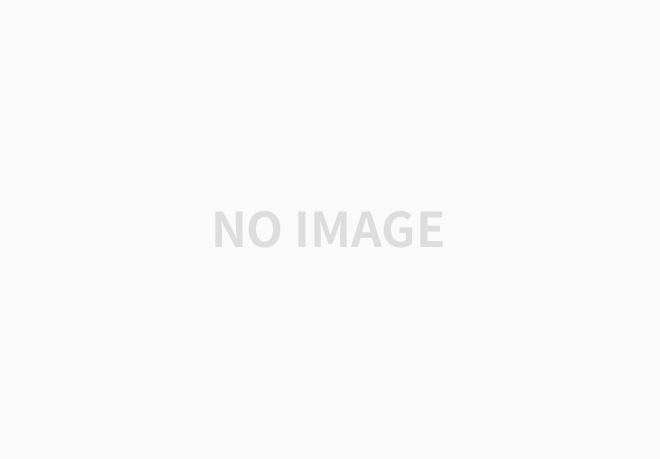
Figure 13. Sediment data
For the upstream boundary condition, it has been input the data of Figure 6 that shows the rating curve of the discharge and the suspended sediment load. And also, the sediment time series has been applied to the downstream boundary condition.
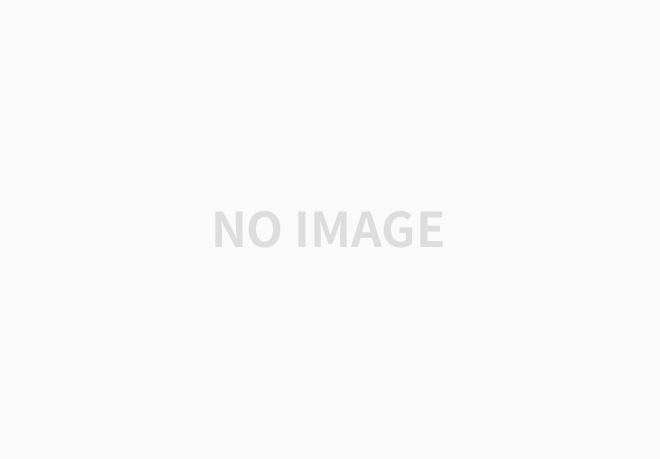
Figure 14. Boundary conditions of upstream and downstream
3.3 Geometric data
Around the middle of October 2014, ISRIP which is the affiliated organization of the WAPDA has been using the section data that was bathymetrically surveyed for the river cross section about 2km near the powerhouse of the Jhelum river, to the geometric data. In addition, the section which was removed the cofferdam of the powerhouse was applied as well. For the roughness coefficient, the value of 0.035 was used and also the value of 0.1 and 0.3 has been applied as the contraction and expansion coefficient, respectively.
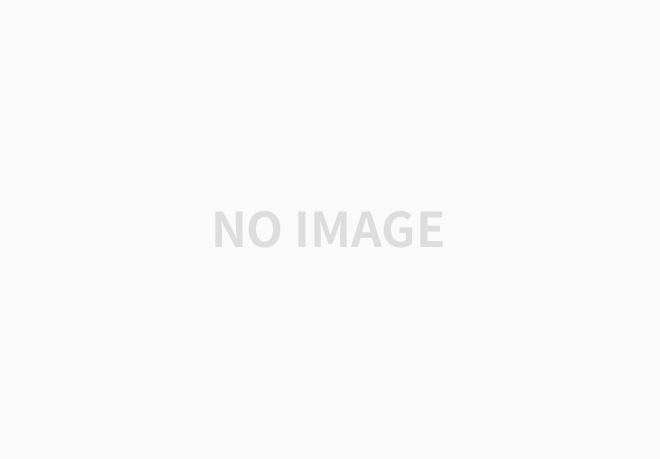
Figure 15. Cross section data
3.4 Result of bed profile in the Jhelum river after 5years
It has been continuously simulated for 5 years from 1 January 2015 to 31 December 2019.
Figure 16 shows the simulated result for 5 years. The black line is the current river bed and it shows that the river regime will be gradually eroded every 1 year. Also, the figure shows that both upstream and downstream, on the basis of the powerhouse, will be eroded because the suspended sediment load in the Jhelum river is relatively small, thus it is considered that the deposition is more predominant rather than erosion. Another reason is that the river bed height of the original powerhouse was much lower than the current river bed height (Figure 10). Therefore, it is possible to assume that the riverbed became higher by any artificial phenomenon (blockage of Jhelum river and install action of the cofferdam, Figure 2). Thus, the phenomenon that river shall be returned to the original status is dominant. In addition, the stream bed degradation is appeared in there, this means the phenomenon that the river bed shall be eroded over the comparatively long-distance and the river bed end slope shall become smaller accordingly.
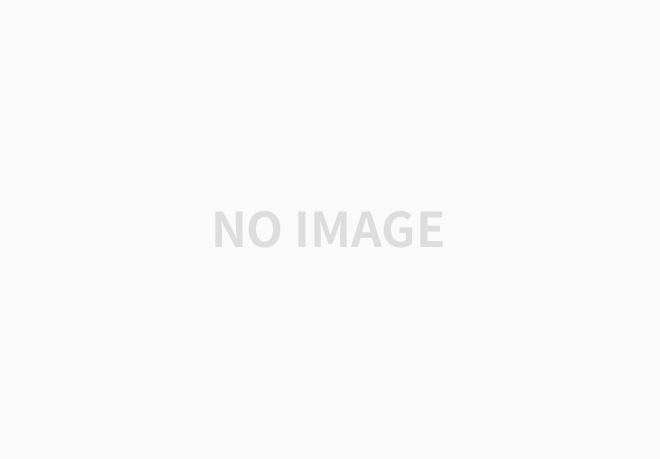
Figure 16. Bed evolution during erosion for average sediment rating curve during 5 years
Figure 16 shows the long cumulative volume change. According to this, about 200,000m3 will be eroded after a year and approximate 520,000m3 will be eroded 5 years later and these will be moved to the downstream. Thus, through this graph, it is possible to predict that the Jhelum river around the powerhouse shall be returned to the river bed status before construction.
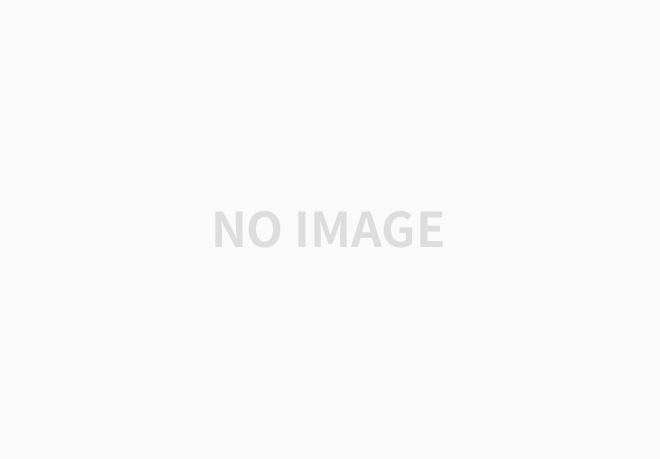
Figure 17. Long cumulative volume change for average sediment rating curve during 5 years (Powerhouse)
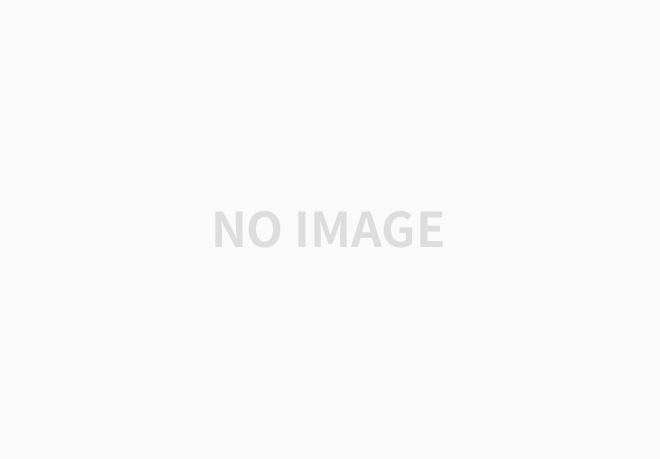
Figure 18. Cross section change for average sediment rating curve during 5 years (Powerhouse)
Figure 19 shows the tailwater rating curve at the powerhouse after 5 years.
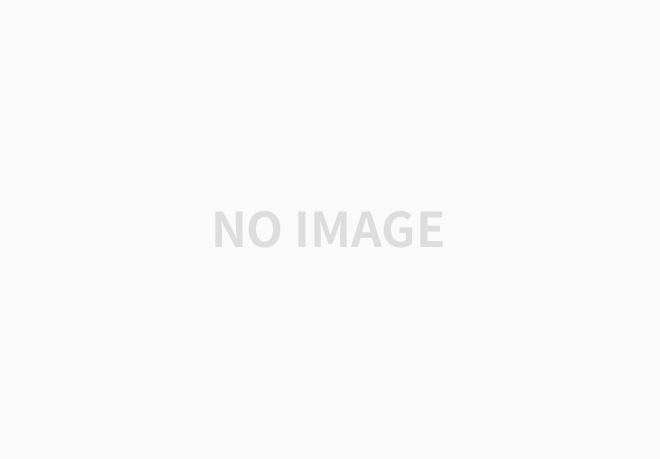
Figure 19. Tailwater rating curve at Powerhouse after 5years
Figure 20, represents the marking at the basic design stage, current river bed and the Tailwater Rating Curve (TRC) from 1 years to 5 years later. According to the Figure 20, it is possible to confirm that the water level in the present TRC is much higher as compared to the basic design stage. This is because that the material was dumped to the left bank in the downstream of the bridge and the width of river cross section was decreased accordingly.
As time goes by, although the stream bed in equilibrium status shall be returned erosion of the river, however, the high status of water level will be maintained even after 5 years between EL.649 and El.651m section. In other words, even though the river will be eroded, but it will not be eroded more than a certain depth. This is because if the narrowed river width will not improve, then the TRC shall be maintained the high status. Therefore, it needs to widen the river width through the method of carrying out the partial mechanical dredging for the river width near the powerhouse. If you see Figure 20, it shows that the water level is rising in the section between EL.650 and EL.653m. Thus, it should expand the main channel below EL.651m of the river.
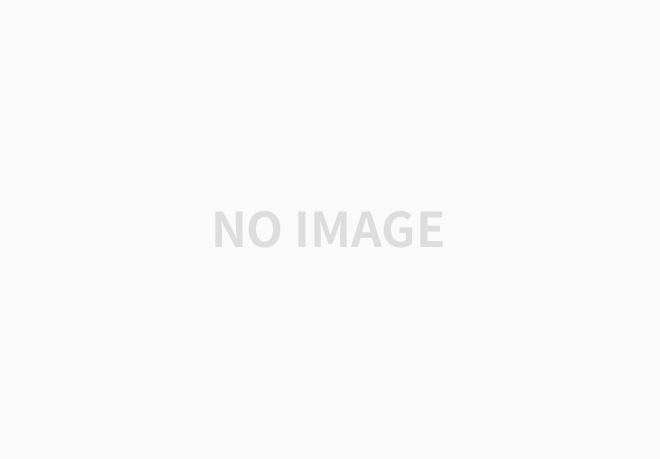
Figure 20. Comparison of the Tailwater rating curve at Powerhouse
Figure 21 is the front view from the access bridge toward the downstream direction. It shows that the width of the river shall be reduced because the left bank is used for the M&E workshop site and also it is used as a partial disposal area. In the long term, the downstream water level shall be decreased due to the erosion in the river bed. However, the partial mechanical dredging should be performed in this area during the dry season just before the completion of this project because the water level in the river has to recover until the water level in the basic design stage at the end of December 2016 when this project will complete.
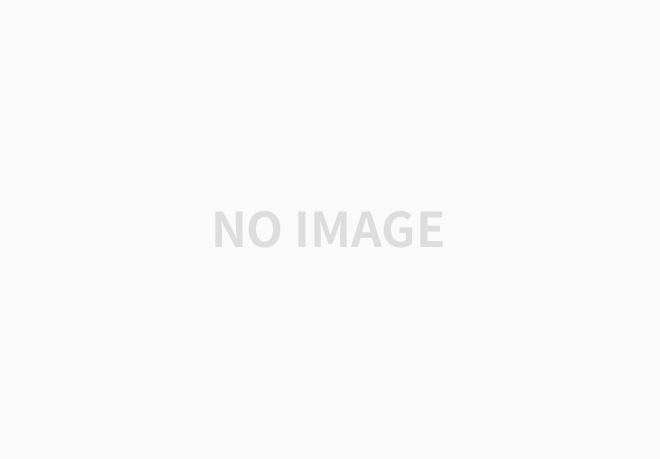
Figure 21. Dumped materials from left bank (View from access bridge)
4. Sediment Simulation of Jhelum River after Dredging
4.1 Dredging Plan
The Dredging Plan has been planned based on the sediment transport of the Jhelum river. The natural river erosion was considered by the force of flow velocity of Jhelum river. Thus, it is planned with the method that minimizes the mechanical dredging.
Figure 22 shows the comparison of the river bed level between the current river bed and around the powerhouse after dredging. The dredging has been carried out from the upstream (No. 3) to the downstream (No. -1) of the powerhouse (+2). That is, the river bed became lower in order to occur the erosion in the river by the natural force.
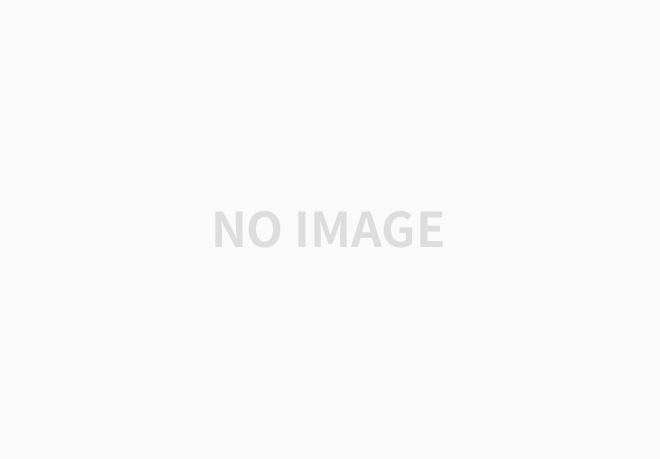
Figure 22. Comparison of Bed profile after dredging
Figure 23 shows the dredging section in the powerhouse section. It has been secured the effective wet section through the dredging up to EL.649m because the river bed has already been climbed compared to the past.
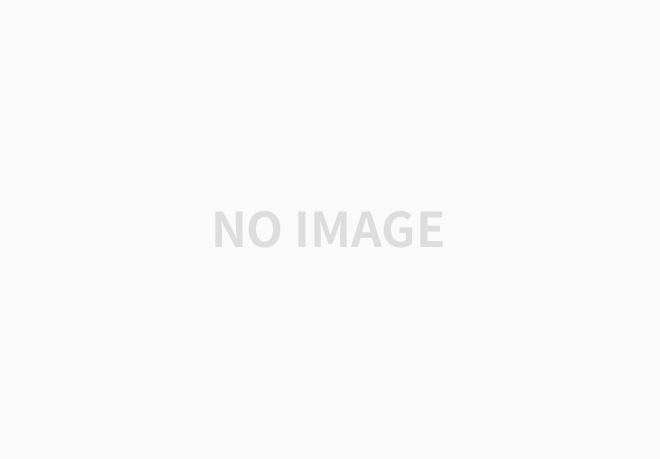
Figure 23. River cross section at Powerhouse after dredging
4.2 Results of Simulation of Jhelum River with Dredging Plan
This is the simulation result during 5 years after dredging work. According to the result, the bed profile shows that the river bed has been eroded more as compared to the bed profile before dredging (compare this with Figure 16). Thus, the river bed erosion has been accelerated due to the dredging work around the powerhouse.
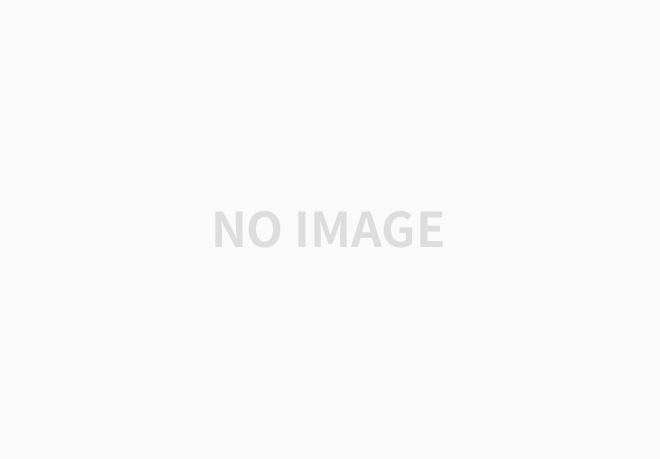
Figure 24. Bed evolution during erosion for average sediment rating curve during 5 years after dredging
Additionally, the amount of the river bed erosion has been increased rather than before dredging. According to the Figure 25, the total amount of the river bed erosion will be about 520,000m3 after 5 years before dredging.On the contrary, after dredging, the total amount of the river bed erosion will be about 620,000m3 after 5 years. In other words, it is resulted that about 100,000m3 of the river bed shall be eroded.
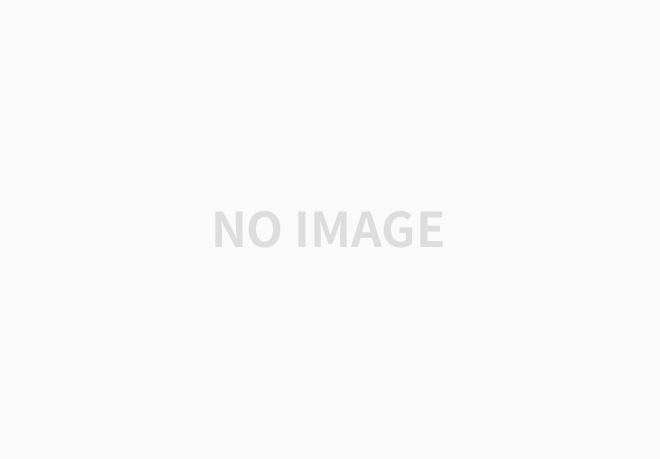
Figure 25. Long cumulative volume change in average sediment rating curve
during 5 years after dredging at Powerhouse
Figure 26 shows the variation of the river bed at the powerhouse during 5 years.
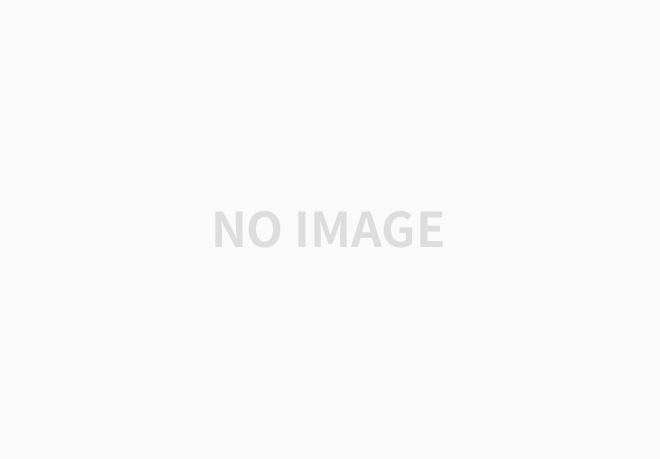
Figure 26. River cross section change with dredging for average sediment rating curve
during 5 years after dredging at Powerhouse
Also, Figure 27 shows the Tailwater rating curve at the powerhouse after 5 years.
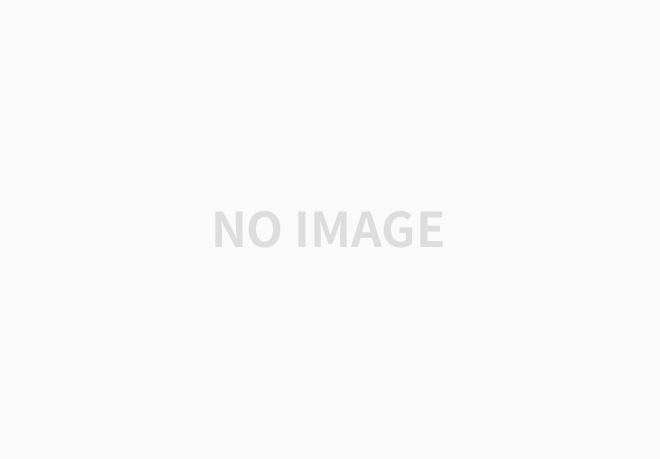
Figure 27. Tailwater rating curve at Powerhouse after 5 years after dredging at Powerhouse
Figure 28 shows the comparison of the Tailwater rating curve after dredging. The water level during the powerhouse operation when it calculates the power generation, is mainly formed at around EL. 650~652m. Of course, the water level is changeable at any time by the inflow discharge of the Jhelum river. For about 500 ~ 1,000m3 of the discharge section in the Figure 28, it shows that this section will be decreased to the water level of the basic design stage 2 years later. Also, for the time between March and July when about 1,000m3 of discharge will inflow, it will be similar to the basic design stage 2 years later, but the water level will be lowered further from 3 years later. This shows that the river bed in the Jhelum river shall be returned to the original river bed level. Therefore, it is judged that it will be beneficial for the power generation because the tailwater rating curve will be lowered rather than the basic design stage from 2 years later (completion time).
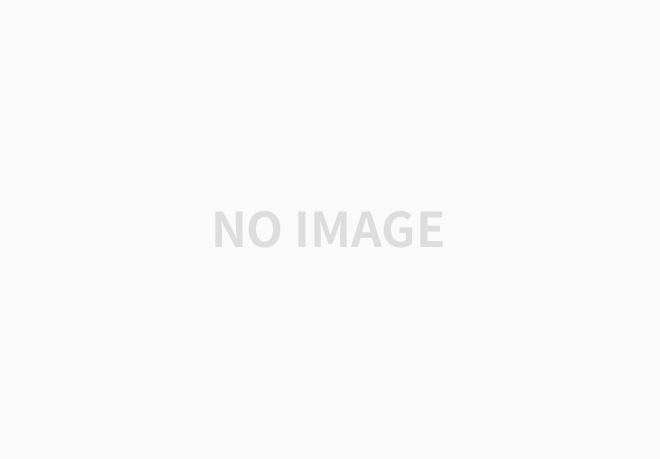
Figure 28. Comparison of the Tailwater rating curve after dredging at Powerhouse
In addition, once the Neelum-Jhelum Project will be completed, the inflow of 300m3/s discharge will be decreased and also the large amount of the sediment inflow will be reduced accordingly. If the dam is constructed at the upstream, that downstream should become a ‘Hungry River’. Thus, the phenomenon that most of the sediment is caught in the upstream dam and there is almost no sediment in the water that is discharged, shall be occurred. Because of this phenomenon, the bottom of the downstream shall be scoured and also the river bed will be lowered.
4.3 Water surface profile
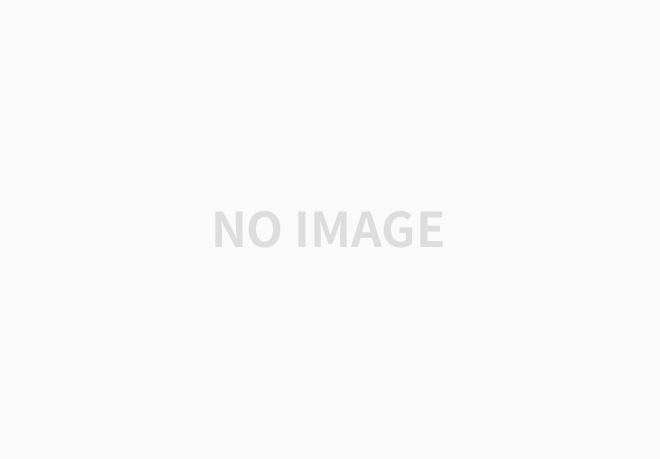
Figure 29. water surface profile after dredging when maximum discharge occur at 18 May every year.
Figure 29, water surface profile after dredging when maximum discharge occur at 18 May Because the water level is below EL.660.0m every year, it shows that it will not affect to the upstream due to the influence of backwater.
4.4 Additional analysis for long-term period
It was conducted to analyze for 30 years long-term period of sediment transport analysis for Jhelum river. In Figure 30, It was showed that bed profile of Jhelum river was changed by every 5 years after dredging. It is expected that the bed profile of Jhelum river could be lowered about 4~5 meters and be stabilized during 30 years.
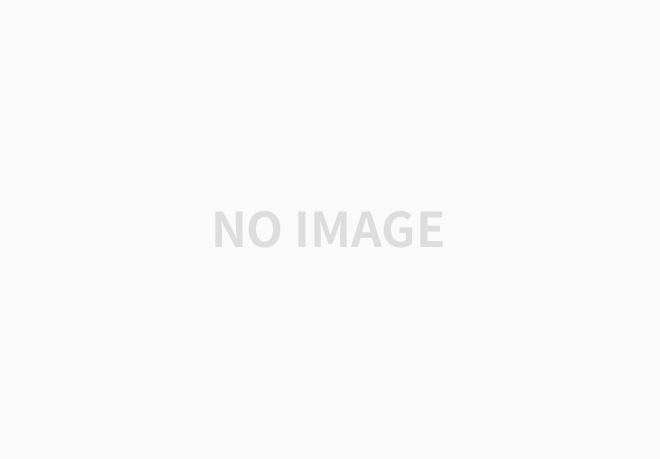
Figure 30. Bed evolution during erosion for everage sediment rating curve during 30 years after dredging
Figure 31 shows the water surface profile oaf a 100 year-return period floods after 1 year of dredging. As shown in the figure below, even if a 100 year-return period floods comes the water level at the powerhouse and bridge is lowered than El.662 m. The powerhoues and the bridge are safe for floods in the present cross section of the bridge.
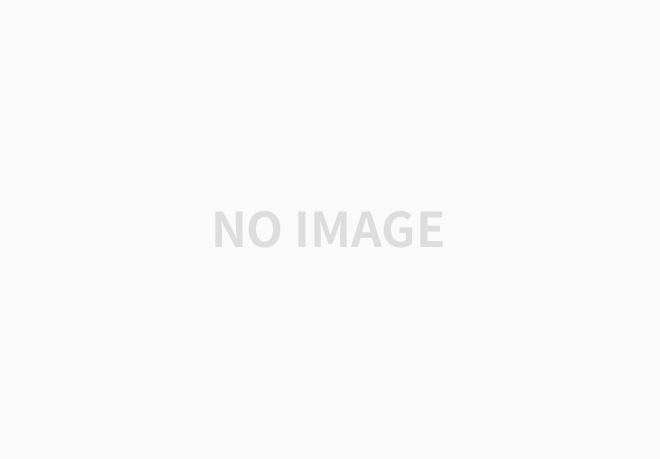
Figure 31. Water surface profile of 100 year-return period floods after 1 year of dredging (Q=8,412 m3/s)
5. The Amount of the Dredging for Jhelum River
The dredging work on the Jhelum river has been performed with the partial removal of the area where is currently used as the M&E workshop site. Even though the dredging is carried out during the dry season, however, it is difficult to perform the dredging work until the middle of the river bed realistically. Thus, the major range of the dredging work has been decided to the left bank.
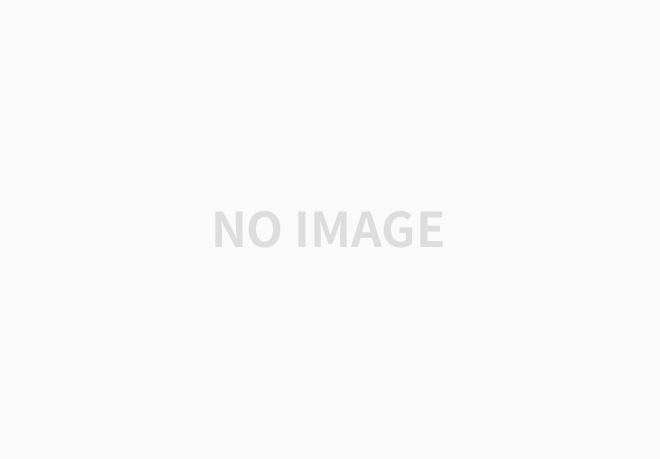
Figure 32. Dredging area in the M&E workshop area
For the dredging amount calculation, firstly, the size of the section was calculated before and after dredging work from No. 3 to No. -1. After that, the dredging amount was calculated by the use of method of average end areas. A s a result, the dredging amount has been calculated as 140,000m3.
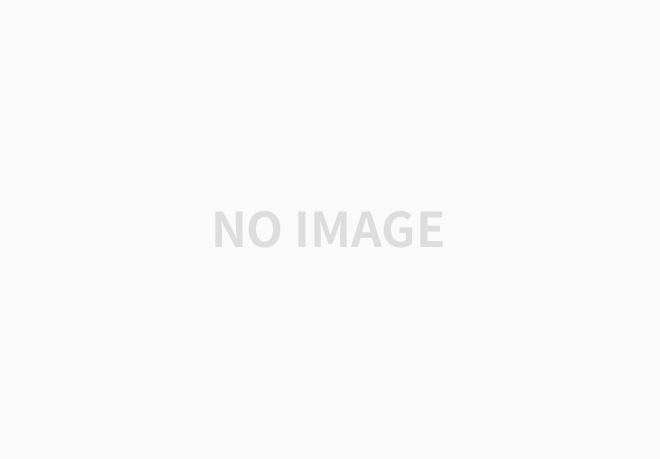
Figure 33. Dredging plan of the Jhelum river
6. Conclusions
◦ The river section has been decreased by the bridge construction during the Patrind Hydropower Project as well as the dumped materials to the left bank.
◦ The riverbed-level gradation has been predicted through the sediment transport analysis of the Jhelum river which was used the HEC-RAS model.
◦ For suspended sediment data between 1980 to 2013 was used and the flow discharge used the mean daily discharge between 1976 and 2012. In addition, for the geometric data, bathymetrically surveyed data of Jhelum river given by the ISRIP on November 2014 was used.
◦ According to the simulation result for 5 years from 2015 to 2019, it shows the stream bed degradation phenomenon.
◦ The amount of erosion in the Jhelum river will be about 200,000m3 after 1 year and also will be about 520,000m3 5 years later.
◦ However, the tailwater rating curve shall be influenced to the power generation because the water level between EL.650 and EL.653m is higher. Therefore, it is required to carry out the partial dredging work to the left bank of Jhelum river.
◦ According to the simulation result after the partial dredging work of the left bank, the erosion amount shall be about 620,000m3 after 5 years. It means that approximately 100,000m3 shall be eroded more rather than before dredging work.
◦ Consequently, the effect of the dredging work is to accelerate the river bed erosion in the Jhelum river.
◦ After dredging, the Jhelum river shall be lowered up to the river bed before construction and also the Tailwater rating curve shall be lowered as well. Therefore, it will have a positive effect to the power generation.
References
1. ASCE, Sedimentation Engineering, ASCE-Manuals and Report on Engineering Practice
2. Chid Ted Yang, 2003, Sediment Transport, Theory and Practice, Krieger Publishing Company
3. Gregory L. Morris & Jiahua Fan, 2010, Reservoir Sedimentation Handbook, version 1.04
4. International Sedimentation Research Institute, Pakista(ISRIP), 2014, Patrind Hydropower Project Bathymetric Survey of Kunhar and Jhelum Rivers
5. Ph.D. Woo, H., 2001, River Hydraulic, Sediment Transport Ch.10
6. Fichtner, 2013, Report on reservoir sedimentation 2nd version
'Tech > 삼안기술지' 카테고리의 다른 글
[21년01월] 5. 아스팔트를 이용한 마찰증대 매트의 기술개발 (0) | 2021.01.12 |
---|---|
[21년01월] 4. HEC-6 모형을 이용한 유사량 공식에 따른 하상변동 민감도 분석 - 낙동강 하류를 대상으로 (0) | 2021.01.12 |
[21년01월] 2. 유기물 부하에 따른 Anaerobic digestion 및 Bio-electrochemical anaerobic digestion의 박테리아 군집 (0) | 2021.01.11 |
[21년01월] 1. 수돗물 깔따구 유충발생 사고의 원인 및 정부 정책방향 (0) | 2021.01.06 |
[제3호] 2021년 1월 제 3호 삼안기술지 (0) | 2021.01.04 |